QMAP Science
We seek to understand the wheels within wheels of our Universe. To many of us, Universe evokes the space and time we're immersed in. But this is not just a passive arena on which all the action takes place: spacetime itself is the key player!
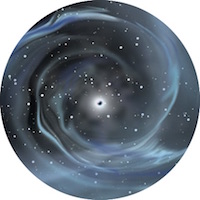
According to General Relativity developed by Einstein one century ago (2015 is the centennial of his fascinating discovery), spacetime curvature manifests gravity; matter and energy actually curve spacetime, even to the point of creating regions of no-return, known as black holes. Remarkably, black holes are far more than merely intriguing astrophysical objects: they underlie our understanding of the universe in a fundamental way, providing invaluable theoretical laboratories which can shed light on a wide class of more familiar systems.
Yet paramount as they are, black holes, and spacetime itself, are constructs defined in a classical setting. Ultimately, the world is quantum mechanical, and the description using the continuum fabric of spacetime ceases to be valid when quantum effects become important.
- What is the underlying nature of spacetime?
- How does it emerge from a more fundamental description?
- For example, why do we see 3 spatial and 1 time dimension (at least on macroscopic scales)?
- How did the Universe get started, and what will be its ultimate fate?
These are some of questions which we are attempting to answer. Developing the correct complete and self-consistent mathematical framework in which to do so -- often dubbed quantum gravity -- is the first step, and has been the Holy Grail of theoretical physics for decades. Although the regime where quantum gravity effects become important does not lend itself to experimental exploration, mathematics comes valiantly to the rescue: the requirement of self-consistency is in fact highly constraining.
Where do we start? One powerful framework is string theory, which is a quantum theory that contains gravity as well as the other fundamental forces of nature. This elegant structure provides a vast edifice in theoretical physics and mathematics, linking previously disconnected concepts and uncovering new ones. But despite several decades of amazing new discoveries, we have understood just a small part of string theory; there is still much to be uncovered.
One intriguing avenue which we've glimpsed recently suggests profound connections between spacetime geometry and quantum entanglement: the underlying concepts may in fact have quantum information theoretic origins.
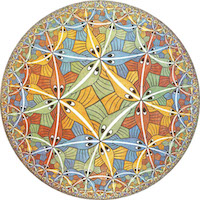
A concrete context whereby to understand this better is the remarkable gauge/gravity (a.k.a. AdS/CFT) duality: string theory, living on a certain spacetime (Anti de Sitter, abbreviated AdS) is physically equivalent to a certain gauge theory (which is a conformal field theory, abbreviated CFT), living in lower dimensions! For this reason the AdS/CFT duality is often called holographic.The gauge/gravity duality has been tremendously successful in elucidating many of the long-standing open problems, by recasting them in the dual language. Yet after almost two decades of active harvesting the fruits of this duality, it still has far more to offer. The main prerequisite in utilizing its potential is understanding of the dictionary between the two sides, which we are still in process of further developing.
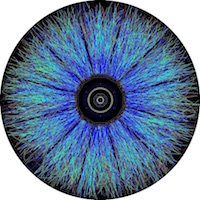
Since the standard model of particle physics is described by a quantum field theory (though not a conformal one, it nevertheless shares many of the universal features with the CFT of interest), we have some modest experimental access to understanding string theory using its holographic dual. Indeed, some systems studied in condensed matter physics and atomic physics likewise exhibit properties which can be understood using holographic techniques.
While a more comprehensive understanding of string theory will enable us to get closer to a theory of quantum gravity, we have also in recent years come to realize that our language for describing quantum dynamics, viz., quantum field theory, may perhaps be reformulated in terms of more simple building blocks.Motivated by the search for organizing principle to explain mysterious relations in certain gauge theory scattering amplitudes, the recently-developed mathematical structure known as the amplituhedron provides an intriguing overarching framework. In this picture, not only spacetime geometry, but string theory and field theory are themselves emergent quantities.
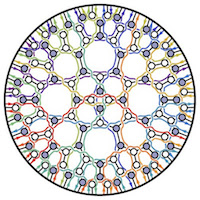
The theme of emergence which permeates many aspects of modern science, in this context suggests that the dynamical objects which we associate physical phenomena with are not themselves fundamental. They should rather be thought of as composite objects which are built out of some basic building blocks. This principle permeates much of modern physics (cf., Dalton's atomic theory) it is just that the turn of the century has revealed to us that the building blocks may be vastly different from the dynamical objects we are used to!
Underlying all of these investigations is an intricate mathematical structure. From a theorist's viewpoint these provide valuable clues on how to best represent the phenomena encountered in an elegant framework. Part of the goal of the QMAP program is to exploit developments in modern mathematics to further our understanding of the physical phenomena.
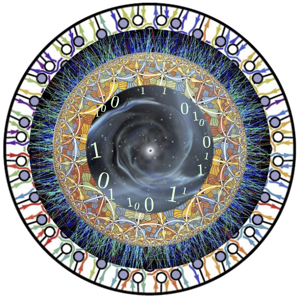